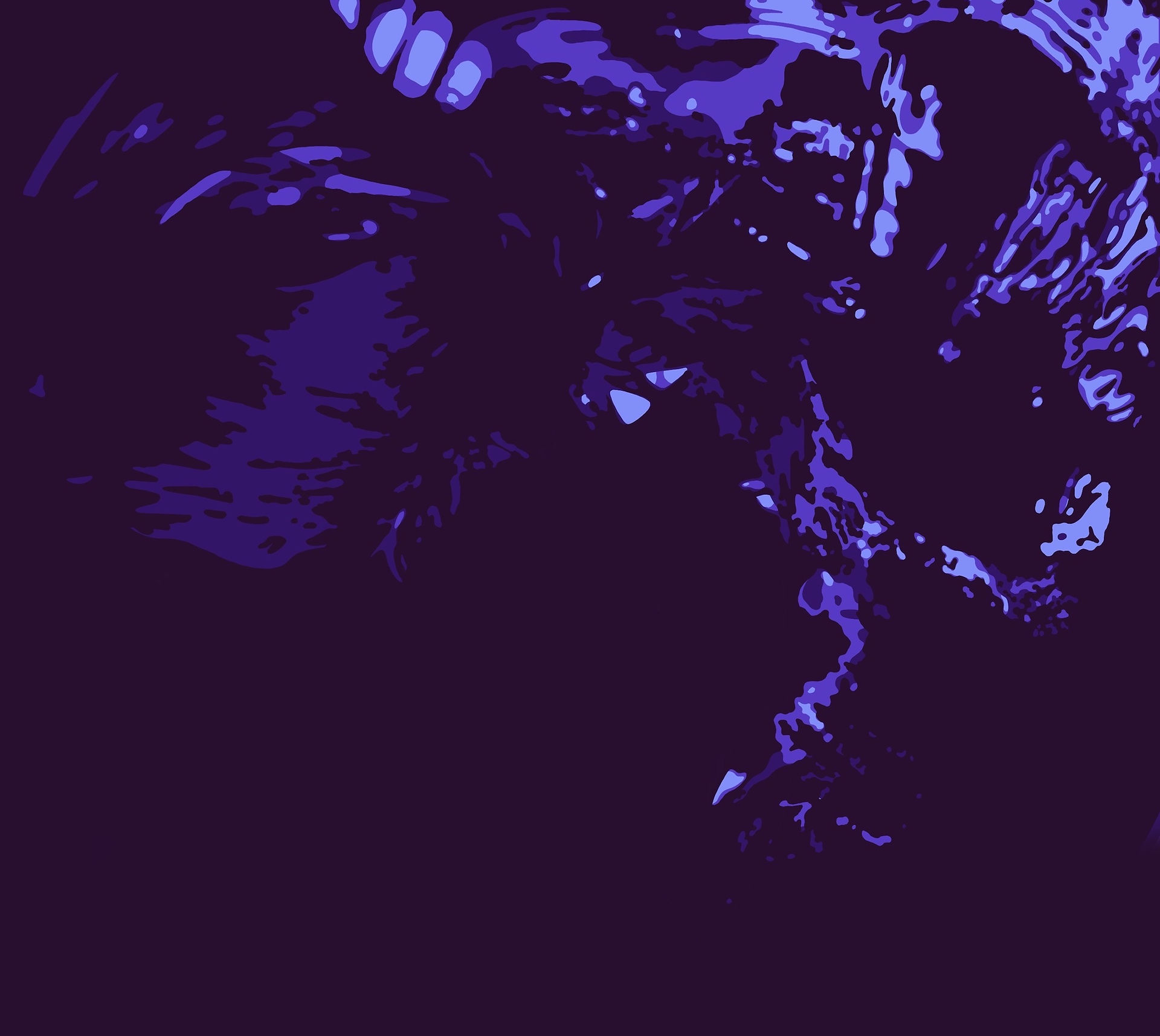
MARS 2030
April 2019 - June 2019
This material science design project was part of a class called DTC. Four team members and I were tasked with coming up with a design that could be used to colonize Mars. More specifically, to design a material that could be used to build rocket launchpads and landing pads in situ on Mars.
SKILLS
Research
Expert interviews, client interviews, literature
Material Design
Thermocalc, phase diagrams, sample design
Fabrication
Die pressing, hydraulic pressing, sintering
Testing
Experimental design, compression testing
Data Analysis
Stress-strain curves, statistical analysis, paired t-test
Collaboration
Delegation, documentation, weekly meetings
PROCESS
Research
Ideation
Fabrication
Testing
Analysis
DESIGN CRITERIA
The material must...
Be able to withstand 1200˚C to 1600˚C for at least 6.6 seconds
Withstand multiple thermal and compression cycles, without fatigue
Withstand at least 2 MPa without fracturing
Use a minimal amount of transported supplies (cargo)
RESEARCH
Earth | Mars | Simulate |
---|---|---|
1x Earth Gravity | 0.375x Earth Gravity | No |
Varies greatly by location | High content Si, Al, Mg | Yes |
14 degrees C | -63 degrees C | No |
-88 to 58 degrees C | -125 to 20 degrees C | Yes |
Primarily N and O | Primarily CO2 | No |
Sintering
-
Turns a powdered material into a solid by compressing and partially melting it
-
Increases material compressive strength
-
Ideally done at 85% of material melting point
Martian Regolith
-
Mojave Martian Simulant-1 (MMS-1)
-
Silica, Iron (II) Oxide, Alumina
-
Melting point: 1200˚C and 1400˚C
-
Melting point must be increased for rocket heat
-
-
The addition of alumina increases MMS-1 melting point
The martian soil needs an additive that will increase its melting point, which alumina does. There are two alumina sources readily available on Mars: kaolinite and feldspar. Kaolinite, an alumina-silicate clay, has a better alumina-silica ratio and will be the most effective additive.

Phase diagram of martian soil, with varying mass percent alumina
FABRICATION & TESTING
Fabrication
-
Mixed 10g of each mixture at 2800 rpm for 30 sec
-
Pressed samples with a hydraulic press
-
Sintered at 1347˚C for 1.5 hrs with a heating/cooling rate of 5˚C/min
-
21 samples total
-
2 compositions: 24 wt% and 34 wt% alumina
-
2 diameters: 6mm, 9mm
-
2 types of thermal treatment: as fabricated, thermally shocked
-
Key issue: height:diameter ratio was inconsistent

Testing
-
Thermally shocked half of samples (2 rounds of 10 sec, 1030˚C - 1080˚C)
-
Compression tested all samples (Sintech 200, Program 331)
-
Measured max. failure load and compression strength
-
Graphed stress-strain curves
Sample Compositions
Alumina wt% | MMS wt% | Kaolinite wt% | Est. Melting Point (˚C) | Sintering Temperature (˚C) |
---|---|---|---|---|
34 | 10.5 | 89.5 | 1577 | 1347 |
24 | 63.8 | 36.2 | 1327 | 1347 |
RESULTS & ANALYSIS
34 wt% Alumina samples had the best compression strength.
Thermal shock had no negative impact on sample performance, and all samples met the compression strength requirement.
Paired t-Statistic Confidence Intervals
(difference in compression strength between 24 wt% and 34 wt% alumina samples)
95% Confidence Interval
9.770 MPa to 23.96 MPa
98% Confidence Interval
7.870 MPa to 25.86 MPa
Neither interval contains 0, so there is most likely a difference in compressive strength between the compositions. We used Protodyakonov's geometry correction to account for inconsistent height:diameter ratios.

Sample compression strengths, compared. The design requirement for compression strength was 2 MPa, which all samples meet.
PHOTOS
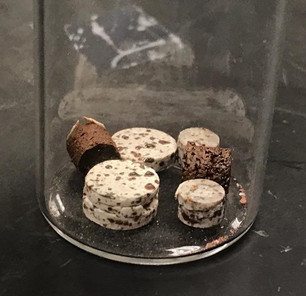
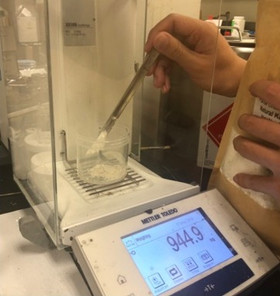

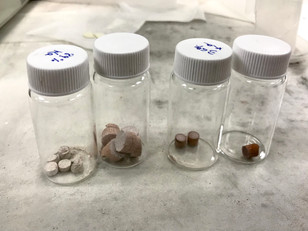
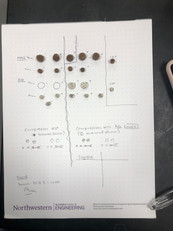
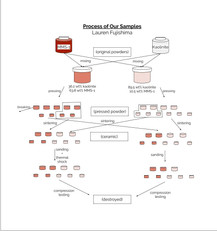
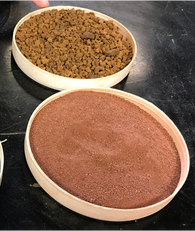
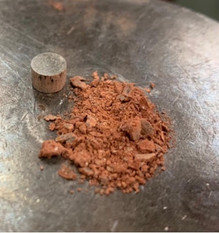

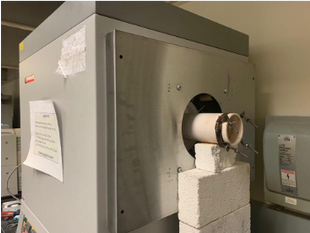

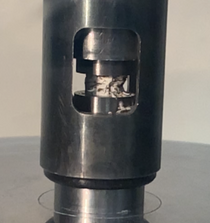
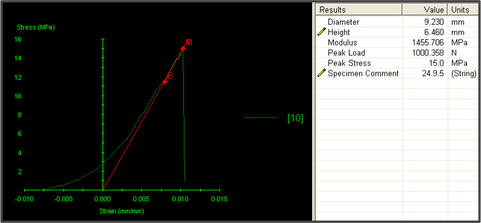
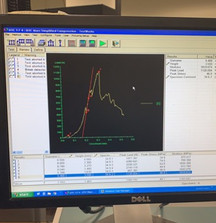